The Tremethick Group - Chromatin and Transcriptional Regulation during Development
Chromatin and transcriptional regulation during development
Research themes
About
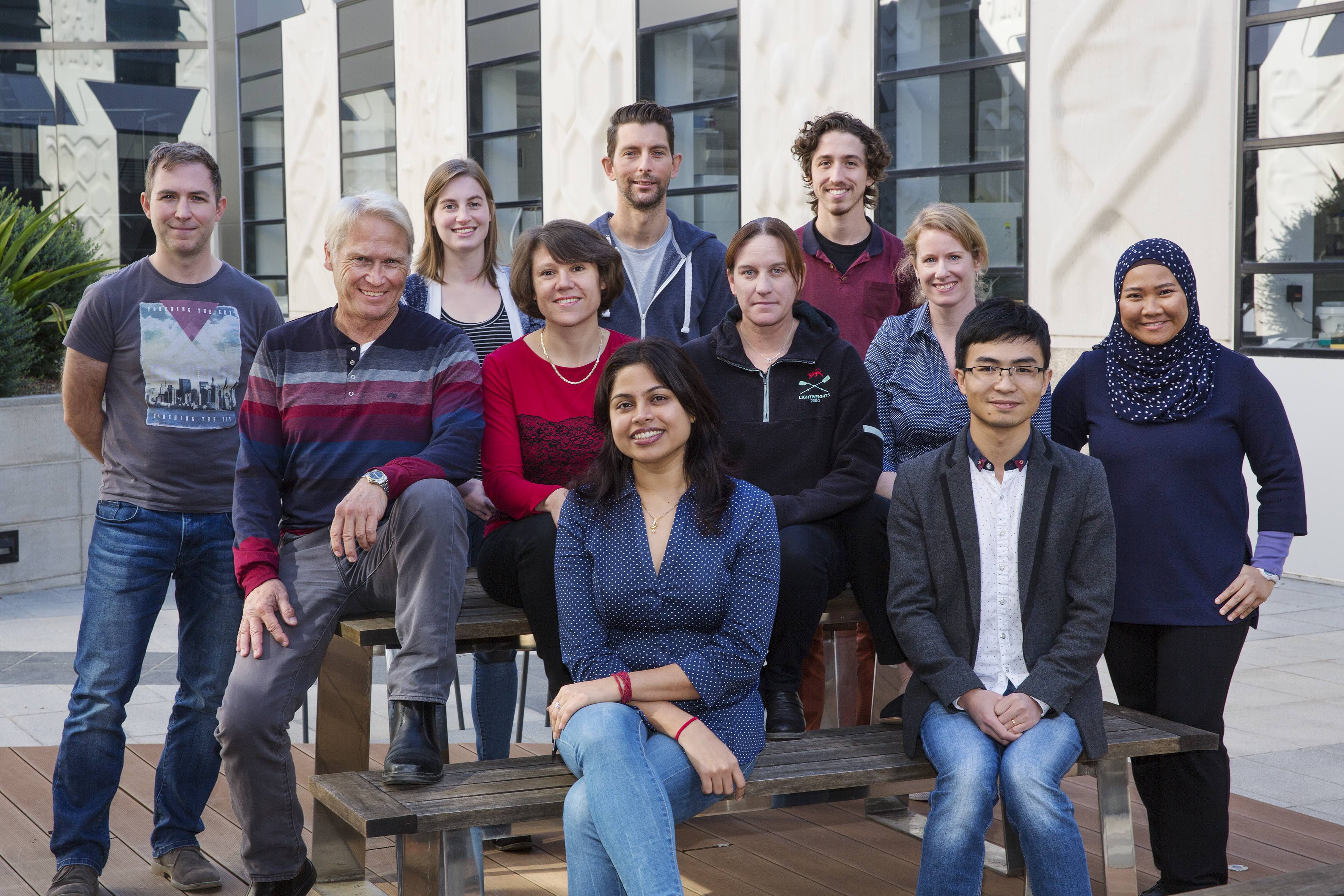
Although it has now been eighteen years since the human genome was completely sequenced, how this genomic information is selectively utilised to direct patterns of gene transcription and pre-mRNA splicing remains poorly understood. However, it has been well established that different epigenetic modifications, such as histone post-translational modifications (PTMs) and the substitution of core histones with their variant forms, play an important role in controlling all stages of the transcription process by modulating the accessibility of DNA. Collectively, this epigenetic-based information at a genome-wide level has been referred to as the epigenome, and there is also now overwhelming evidence that it is the epigenome that co-ordinates the gene expression pattern unique to each different cell type in the human body.
Our work has demonstrated that the exchange of the core histone H2A with its variant forms, in particular the essential histone variant H2A.Z, represents one of the most profound and crucial epigenetic processes that is required for early metazoan development, chromosome organisation and inheritance, as well as regulating promoter chromatin architecture to maintain or change cell fate. Mechanistically, we demonstrated that H2A.Z, and other types of H2A variants, performs these crucial functions by directly regulating the extent of chromatin compaction. Recently, we have also found that histone H2A variants are located at intron-exon boundaries to regulate pre-mRNA splicing, which is critical for male fertility and brain function. Moreover, we have found that the mis-incorporation of histone H2A variants into chromatin plays an important role in the progression of cancer.
To further understand the role of the epigenome, including the role of histone variants, in regulating genome function, we have established long-range genome mapping and computational 3D genome modelling approaches, which revealed how active and inactive genes are packaged and segregated into different 3-dimensional compartments within a chromosome during the differentiation of human stem cells. However, despite these advances in knowledge, key questions remain unanswered:
1) how does the local ‘nucleosome beads-on-a string’ structure of chromatin fold into large spatially organised 3D structures and the role of histone variants in this process?
2) What is the mechanism of how histone variants regulate RNA processing, and its link with brain function and cancer? and
3) How does the epigenome co-ordinately regulate patterns of gene expression at a global level to control cell function?